One model to rule them all: Thermal-death-time model reconciles heat tolerance measurements in ants
In the recent paper “Thermal-death-time model as a tool to analyze heat tolerance, acclimation, and biogeography in ants” by Quentin Willot, Michael Ørsted, Christian Damsgaard, and Johannes Overgaard published in Myrmecological News, the authors investigate thermal-death-time (TDT) models to describe heat-tolerances in several European ant species. They also revealed that soil surface temperatures are poor predictors of species’ heat tolerance. These findings show that TDT modelling offers strong conceptual benefits for integrating heat-tolerance metrics resulting from various methodologies. However, most of the interspecific data on heat tolerance are already captured in the simpler, more commonly used dynamic assays. Here, Jelena Bujan highlights the main points of the study.
A View by Jelena Bujan
The featured image of Aphaenogaster senilis was taken by João Coelho, licensed under CC BY 2.0. No changes were made to this picture.
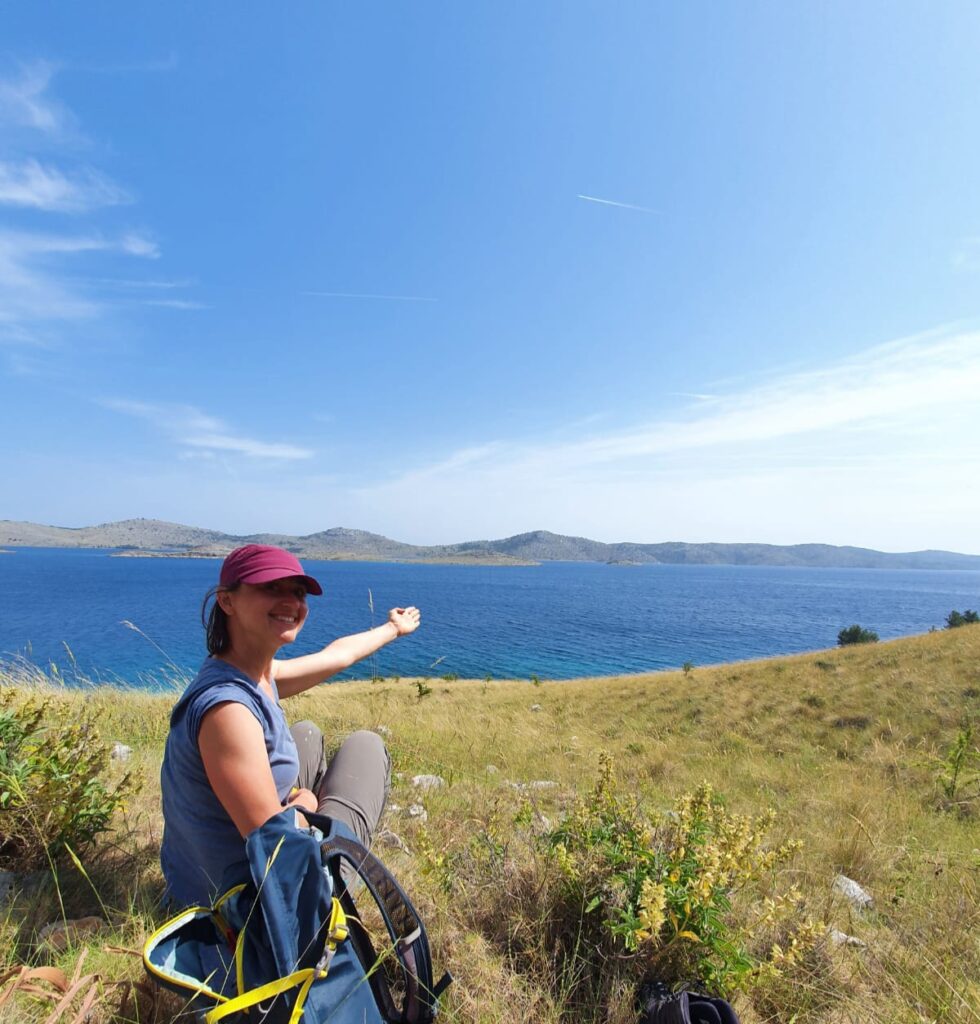
Ant heat tolerance closely regulates food intake important for colony growth and development. The most common way to determine ant heat tolerance is by measuring critical thermal limits — temperatures at which animals lose voluntary muscle control. Yet, the use of critical thermal limits is often criticized as they represent the extremes of organisms’ thermal tolerance and there is no standardized method for measuring them. Despite these criticisms, research on ant thermal tolerance has skyrocketed in the last decade (Roeder et al. 2021), largely because critical thermal maxima (CTmax) are easy to measure and there is a desire to understand ant resilience under global warming towards predicting the winners and losers of climate change. While each method for measuring CTmax has its own merit, there is currently no direct way to compare the results acquired with different protocols (e.g. between static vs. dynamic ramping assays or between different rates of temperature increase).
Willot et al. tackle this methodological can of worms in an extensive study of heat tolerance in 13 European ant species from the two most diverse subfamilies: Myrmicinae and Formicinae. With a combination of methodological comparisons, acclimation experiments, and macroecological approaches, the authors find that the thermal-death-time model has the potential to bridge the methodological gap between different protocols. The experimental part of the study focused on ant species from two climates at the extremes of European temperature and precipitation gradients. One location was in a Mediterranean climate with thermal specialists like Cataglyphis piliscapa, and the other was in a hemiboreal climate with cold specialists like Leptothorax acervorum. For each species, the thermal-death-time model was fitted based on survival time measured at six different temperatures. Parameters of thermal-death time models were able to predict dynamic CTmax regardless of the ramping rate (0.1 vs. 0.25 °C/min), and static CTmax regardless of exposure duration. This makes the thermal-death-time model approach extremely powerful, especially when trying to unite methodologically different studies for global comparisons.
Another useful feature of the thermal-death time models is the ability to calculate a coefficient of thermal sensitivity to temperature change. Aphaenohaster subterranea was the most thermally sensitive species, while the least thermally sensitive ant was surprisingly another Myrmicine with low heat tolerance – the European fire ant (Myrmica rubra). For the same temperature increase, the survival time of A. subterranea was reduced more than M. rubra. The high thermal sensitivity of A. subterranea might be concerning as this species is an important seed disperser, a service that could be disrupted by even slight temperature increases. At the other end of the spectrum, the least thermally sensitive M. rubra is a successful invasive species outside of Europe, perhaps suggesting that lower thermal sensitivity is beneficial when one is transferred to novel climates.
But CTmax and thermal-death-times are likely not fixed. In ants, thermal acclimation – reversible change in thermal limits — of adult workers can happen within 7-10 days after being exposed to new temperatures. This was not the case for the six species acclimated in this study to either cooler temperatures (17 °C) or to warmer temperatures (30 °C). Four of these species are in the genus Lasius (L. niger, L. emarginatus, L. myops, L. flavus) and the other two in the genus Aphaenogaster (A. senilis, A. subterranea). Low acclimatization in subterranean ants such as L. flavus and L. myops is unsurprising as they are adapted to more stable ground temperatures. However, the black garden ant (L. niger) loves disturbed environments and is considered alien in some areas. So, it is often found in urban areas which experience variable climates. The canopy ant L. emarginatus also experiences a more variable climate compared to the understory ants. Given that, one would predict a more flexible CTmax and a higher acclimation potential for both species, which was not the case. All tested species live in cooler climates where cold temperatures might still pose a stronger selective pressure than heat, so their acclimation ability might be higher in regards to their critical thermal minima.
Finally, the study examines heat tolerance in the context of ant distributions. At the continental scale, none of the climatic variables from a new soil temperature database (Lembrechts et al. 2022) could predict thermal tolerances (dynamic CTmax, static CTmax, and thermal sensitivity). We found the same absence of CTmax vs. temperature relationship in a set of North American ant communities when using macroclimatic data based on air temperatures (Bujan et al. 2020). The lack of relationship in heat tolerance and climate suggests that ants might be adapted to local microclimatic conditions that are captured at finer resolutions than 1-km2 and should be the next step when examining the relationship between environmental variables and physiological adaptations.
Thermal-death-time models are a powerful tool in bridging the gap between heat tolerance studies measured with different protocols. One downside of the approach is a much longer protocol which requires a larger number of workers to determine a thermal-death-time model for each species. We yet need to find out how these models vary within species across populations in different climates. Using extensive experimental approaches for widely distributed European species, Willot et al. provide insight into the thermal sensitivity of ants and lend support to the fact that CTmax measured with a reasonable temperature increase is not all that bad, and static and dynamic protocols yield comparable data. Considering the rise in studies on ant heat tolerance, this study is a welcome and timely step forward towards disentangling the complex methods that myrmecologists use to study ant ecophysiology.
References
Roeder, K. A., D. V. Roeder, and J. Bujan. 2021. Ant Thermal Tolerance: A Review of Methods, Hypotheses, and Sources of Variation. – Annals of the Entomological Society of America 114: 459–469.
Lembrechts, J. J. et al. 2020. SoilTemp: A global database of near-surface temperature. – Global Change Biology 26: 6616-6629.
Bujan, J., K. A. Roeder, K. de Beurs, M. D. Weiser, and M. Kaspari. 2020. Thermal diversity of North American ant communities: Cold tolerance but not heat tolerance tracks ecosystem temperature. – Global Ecology and Biogeography 29: 1486-11849.
Recent Comments